BIOSCIENCE Studies related to genetically modified mice
Production of genetically modified mice/rats
Genome-editing technology
Genome-editing technology (ZFN, TALEN, and CRISPR/Cas9, etc.) has common characteristics such as that genetically modified animals can be prepared by injecting RNA into a fertilized embryo.Enzyme with nuclease activity in the targeting site causes Double Strand Break (DSB).When DSB occurs, repair will be conducted by either NHEJ (non-homologous end-joining) or HR (homologous recombination).NHEJ simply binds where DSB occurred, which will be the main repair mechanism during DSB.However, insertion or deletion mutation (indel mutation) of several to several dozen bases may be seen during binding.Knockout of the target gene will be enabled by introducing frame-shift mutation using this repair error.In HR repair, there is a correct sequence as a template which enables an accurate repair, unlike NHEJ.Usually, HR repair is conducted with a sister chromatid as a template. However, by injecting donor DNA, which includes a sequence (homologous region) near the targeting DSB introduction area, with RNA, HR repair will be conducted with donor DNA as a template.In doing so, by applying point mutation to the donor DNA, accurate introduction of point mutation within the genome will be conducted to prepare a model animal.In addition, by using HR, loxP sequence and large size GFP (Green Fluorescent Protein) gene may be introduced (Figure 1).
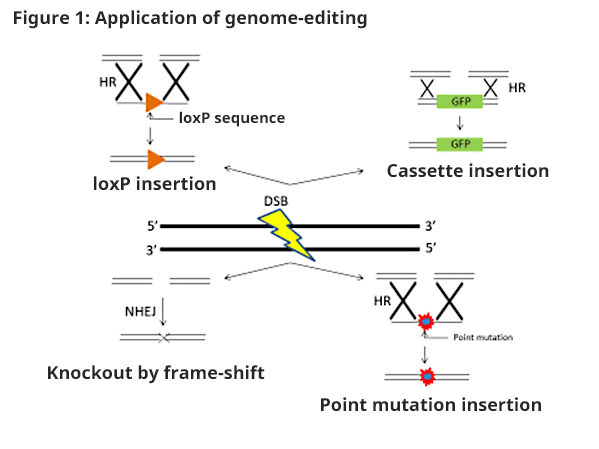
Using this technology, preparations of gene altered organisms are being reported even in organisms with difficulty establishing ES cells. In addition to the preparation of genetically modified animals, gene treatment and various utilizations are also being expected. The characteristics of the 3 types of genome-editing technologies are shown below (Figure 2).
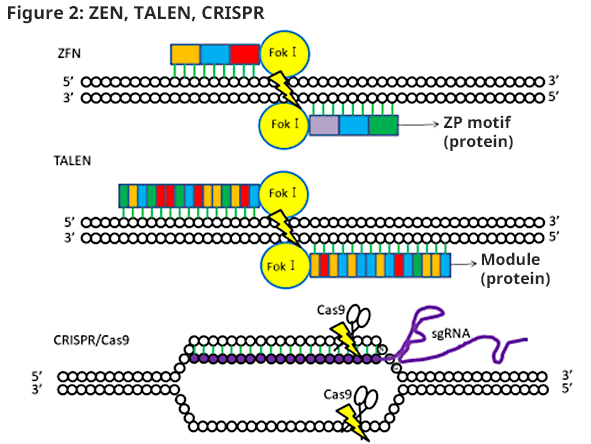
- ZFN
- ZFN is a first-generation genome-editing technology. In 1996, it was reported that DSB was induced at the target DNA by artificial chimera protein with ZF motif, which recognizes 3 bases, and its end binding with Fok I nuclease, creating heterodimer at the target area. One ZF unit recognizes 3 bases, and 3 to 6 units in combination will recognize 9 to 18 bases. The targeting sequence will be recognized by designing a sense chain and antisense chain sandwiching the spacer area of the 5 to 6 base pairs (Figure 2).
- TALEN
- In 2011, it was reported that the second generation of TALEN was developed, which changed the DNA-binding domain of ZFN to TAL effector which is in Xanthomonas genus, a plant pathogen bacteria. When TAL effector binds to DNA, 1 module recognizes 1 base. TALEN, like ZFN, will prepare a sense chain (recognizes 15 to 20 bases) and antisense chain (recognizes 15 to 20 bases) by sandwiching the spacer.
- CRISPR/Cas9
- In 2013, a third-generation genome-editing tool using the CRISPR/Cas system, which acts as prokaryotic acquired immunity, was developed. CRISPR/Cas system breaks foreign DNA, which enters via phage infection and conjugation, to take in to the intrinsic genome. When re-entering, foreign DNA will be recognized using RNA obtained as a result of the first DNA transcription result, and a cut will be performed by CAS nuclease. Unlike ZFN and TALEN, RNA will detect targeting DNA. The only things to prepare are gRNA (guide RNA) with a target sequence and complementary sequence and mRNA of Cas9 protein with nuclease activity. In ZFN and TALEN, the DNA-binding domain and cutting domain must be prepared as a fusion protein and require a complex vector formulation; whereas, the CRISPR/Cas system can formulate tools easily just by changing the base of the corresponding target sequence. Note that 3 bases (NGG) called PAM (protospacer adjacent motif) are needed near the recognized base (20 base), and the degree of freedom in target sequence determination will be lowered; however, genome-editing activity is higher compared to ZFN and TALEN.
Due to its convenience in terms of genome-editing activity, and because the editing tool can be prepared at a low cost, it is widely used in culture cells, mouse, rat, swine, and monkey.
Challenges
These highly versatile methods have several remaining challenges. Some of these challenges and their measures are shown below.
- Challenge 1. Mosaic
- In the case of genetically modified animal preparation using genome-editing technology, RNA will be injected into the pronucleus embryo; however, a fertilized embryo develops and if nuclease cutting of the target sequence occurs in a two-celled embryo and four-celled embryo, mosaic animals with various mutations may potentially be born. As a measure, in simple knockout animal preparation, rather than conducting detailed testing with F0, it is considered to have a more definite result by mating F0 animal and wild type animal to prepare F1 with objective mutation, then confirm gene mutation.
In KO animal preparation, when creating F1 generation from mosaic animals, the fact that several mutant strains will potentially be established would be more of a benefit than a challenge.
- Challenge 2. Off-target effect
- Special caution when using the CRISPR/Cas9 system must be taken because the number of bases recognized by gRNA is 20 bases is short and may cause cutting of a similar target sequence. This is called the off-target effect. As a realistic measure, using guide RNA designing software such as CRISPRdirect, selecting a target sequence with as less similar a sequence as possible and changing the off-target site to wild type by mating with wild type animals may be considered. Moreover, there is a reported method that off-target effect is decreased by increasing the recognized sequence to 40 bases by using Cas9 nickase and 2 gRNA.
Example of genetically modified animal preparation in KAC
Example of genetically modified animal preparation in KAC
- Preparation of ApoE knockout mouse
- ApoE (Apolipoprotein E) knockout mouse has already been prepared using ES cells, and its phenotype is being analyzed in detail; therefore, we’ve decided to use ApoEgene as a target because verification of knockout mouse preparation is easier.
Ninety-three embryos (C57BL/6JJcl-derived) after RNA injection were transplanted to pseudo-pregnant mice and 39 offspring were obtained (F0)(42.0%). Past reports have shown that when ApoEgene is destroyed, the blood total cholesterol (TC) value will rise. TC value was measured in 6-week old F0; 27 out of 39 animals (69%) showed a more than 4-fold increase of C57BL/6JJcl (Control: wild type) 55 mg/dL (220 mg/dL) (Figure 3).
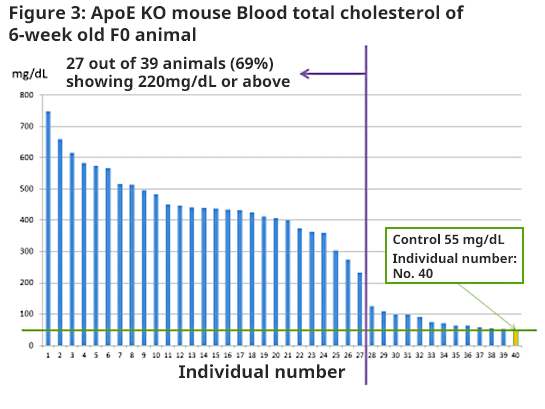
DNA sequence testing was conducted in all F0 animals (39); 2 or more types of mutations were detected in 37 animals (95%), 1 type of mutation and wild type sequence was detected in 1 animal, and wild type sequence only was detected in 1 animal.
Two or more types of mutations were seen in 37 animals, and a phenotype with a high TC value was seen in 27 animals. Animals which did not show a phenotype with a high TC value despite mutations were animals with indel mutation seen in multiples of 3. Even indel mutation was seen in multiples of 3, but it does not result in frame-shift mutation; therefore, it may have not shown a phenotype with a high TC value. Variability in TC value is observed (Figure 3); F0 animal mosaicism is considered to be one of the reasons.
- Preparation of tyrosinase knockout rats
- Tyrosinase gene is a gene which determines coat color; it is known that an albino will be created if both allyls are deficient. Because knockout condition influences the coat color, the presence of the gene mutation may be checked visually; therefore, we attempted to prepare tyrosinase knockout rat. Pronucleus embryo was sampled by mating male DA (colored) rat and female Wistar (albino) rat; then, gRNA and Cas9 mRNA were injected. Fifty-eight embryos after RNA injection were transplanted to pseudo-pregnant rats and 29 offspring were obtained (F0) (50.0%). Three animals were mosaic animals in coat color; however, the other 26 animals were all albino (Figure 4).
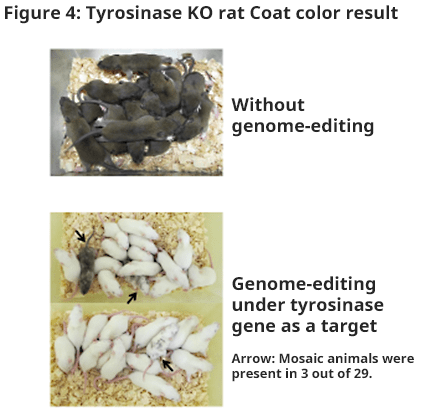
- Preparation of point mutation-induced rat
- Albino rats are known to have point mutation in tyrosinase gene causing albinism (Figure 5, 6).
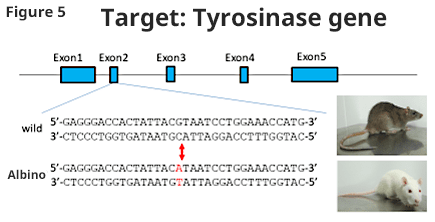
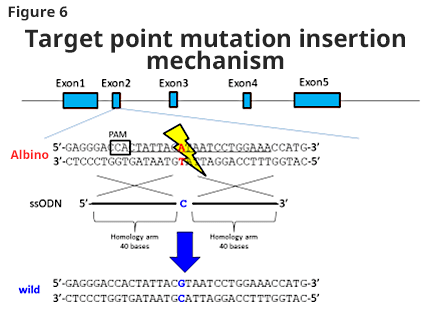
We tried to obtain a colored animal by returning the point mutation to wild type. Pronucleus embryo was sampled by mating male F344 (albino) rat and female F344 (albino) rat; then, gRNA, Cas9 protein, and ssODN (single strand oligo DNA) with wild type tyrosinase gene sequence were injected. Fifty-six embryos after injection were transplanted to pseudo-pregnant rats and 26 offspring were obtained (F0) (46%). Among those animals, 2 animals (7.7%) became colored, and the introduction of point mutation was successful (Figure 6).
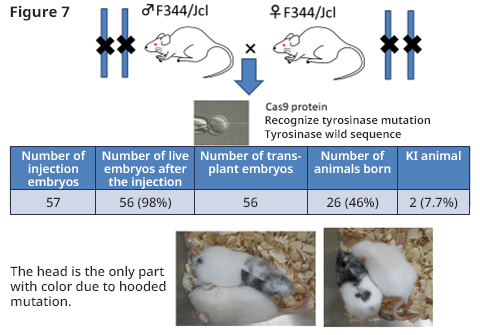
- New genome-editing tool Cpf1 (CRISPR/Cas12a)
- As a new genome-editing tool, we focuse on Cpf1.
CRISPR-Cpf1 classified as Type V CRISPR-Cas system, as with CRISPR-Cas9 classified as Type II CRISPR-Cas system, is constructed of a combination of guide RNA and nuclease; however, guide RNA does not include tracrRNA. Moreover, note that PAM sequence of Cas9 is NGG, whereas, PAM sequence of Cpf1 is TTTN.
In addition, the DNA cutting end of Cas9 is blunt, whereas, that of Cpf1 is protruding.
Regarding cutting efficacy, Figure 8 shows the result of KO mouse preparation being attempted on tyrosinase gene as a target against C57BL/6J Jcl mouse-derived fertilized egg.
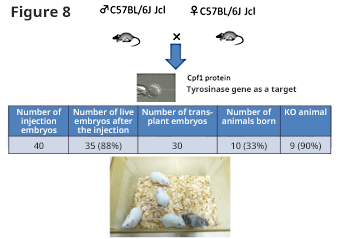
As in Cas9, high genome-editing efficacy was also shown in Cpf1. In addition, high genome-editing efficacy data was also shown in rats.
We are considering an introduction of point mutation, introduction of large size gene, and preparation of flox (conditional knockout, CKO) animals by using Cpf1.
Finally, in preparing genome-editing mouse/rat, CRISPR/Cas9 has conflicts due to its patent, whereas, the basic patent for Cpf1 is owned by the U.S. Broad Institute, which makes it easier to use.
[ close ]